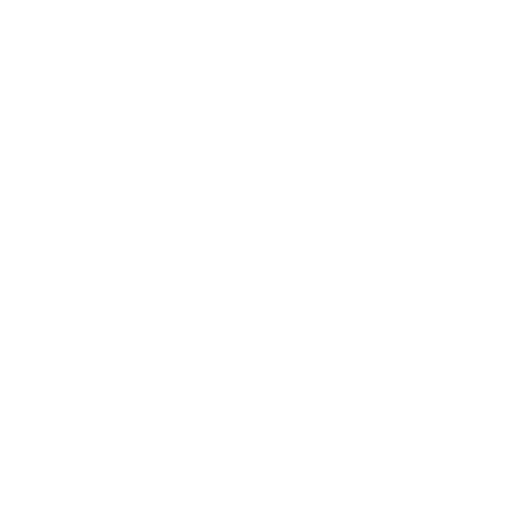

Introduction to Reversibility
Reversibility, the realm of thermodynamics, refers to the characteristic in regards to a particular process that can be reversed. Most importantly, the system should be restored to its primary state without leaving any effect on the other systems which were involved. In simpler terms, the meaning of irreversible is a process that can be reversed without leaving any trace on the surroundings. Irreversible in the process can be owed to the interaction of that system with other processes. An ideal example of this process would be the singular swing of a frictionless pendulum.
What is Reversibility Meaning as Per Scientific Terms?
In scientific terms, the reversibility meaning is determined to be the net heat and work exchange between the system and surroundings (for original + reverse process) is zero. These processes take the least amount of work in the realm of work consuming devices. In proportion, they give the maximum output, owing to which they produce ample interest as they function in an optimum manner. They also act as a highlight to reflect upon the theoretical limits to corresponding irreversible processes. Reversible processes also reflect upon the definition of the second law efficiency for actual processes, which is related to the degree of approximation in regards to the corresponding reversible processes.
In practical terms, it would take an infinity for the whole reversible process to complete itself, thus owing to this contingency, perfectly reversible processes are impossible. However, there is a practical solution to it, which provides us with the same result. So if the system which is going through the changes reacts much faster compared to the change which is being applied to it, in such a rare case, the change caused due to reversibility would be negligible, in other words, not noticeable.
[Image will be Uploaded Soon]
Example of Reversibility
In an optimum reversible process, the energy exerted from the said work being done by the system would be optimum. In other words, to understand the example of reversibility, it would be maximized. The heat being exerted the said performance of the process would be nil to reach this maximum output. But replicating the same in reality is not physically possible, as heat exerted from the performance of the said process cannot be fully converted into work, and some of the heat will always be lost.
This heat will be generally lost to the things surrounding the process. But there are some cases in which the theoretical basis of this proposition does stand true. For instance, in the process of isothermal expansion of an ideal gaseous particle in a piston-cylinder arrangement, this does stand true as no heat whatsoever is lost in the process. In scientific terms, the net change in the composite entropy of the whole system and the surroundings revolving around the process will be zero.
The usage of this process is mainly evident in the creation of heat engines for large commuting vehicles like chartered flight and freight trains. Since it helps minimise the heat lost from the process, it aids the designers in manufacturing the requisite engines, giving the maximum output by reducing the heat exerted from the process. For instance, if an engine gives out less heat, then in one cycle, the energy put into the process will be put to use for the functioning of the vehicle, and less energy will be wasted in the form of heat. The main source through which heat is lost friction is that a certain amount of friction is inevitable as in any process. Thus even in the ideal example, the product used for charging the whole arrangement was gaseous in nature, as negligible to no heat is lost in processes surrounding gaseous particles.
Nicholas Tesla was one of the first pioneers who actually applied this in their work. The Tesla Turbine invented by him had disks that revolved for fastening the said machine to its respective shaft, which was in turn operated by an engine. If the whole process was reversed, meaning, if the turbine’s operation was reversed, the same disks acted as a pump. Another common day example of a reversible process can be seen in the functioning of a reservoir. Heat transfer between a reservoir and a system is an externally reversible process if the outer surface of the system is at the temperature of the reservoir.
[Image will be Uploaded Soon]
FAQs on Reversibility
1. What is the Meaning of the Irreversible Process?
Ans: In scientific terms, if a process is not reversible, then the same is termed irreversible. In thermodynamics, the state of a certain system surrounding and surrounding of the system cannot be totally restored to its initial graph. Some of the other minute changes occur due to the surroundings of the process, at the expense of the energy initially spent in the process. A system as a standalone unit can still go back to its initial state.
However, it is nearly physically impossible to revert the surroundings back to their initial condition. In a sense, reversible processes tend to serve as physical boundaries which can be achievable by the corresponding irreversible processes.
The loss of energy can occur due to a plethora of reasons; some of those reasons are listed below, friction, unrestrained expansion, mixing of two fluids, heat transfer across a finite temperature difference, electric resistance, inelastic deformation of solids, and chemical reactions.
2. List Down Some Examples of Irreversible Processes.
Ans: Most natural processes which are ingrained in the functioning of nature are irreversible. There is an interaction between molecules and these molecules through a certain system brought from one thermodynamic state to another in all-natural processes. For the configuration of the energy, some transformational energy is bound to be consumed. During this whole transformation, there is bound to be some loss of energy in the form of heat. This energy coming from heat will be dissipated due to the complex intermolecular collisions and frictions. Thus even if the whole energy is reversed, there still is some wastage of energy.
The difference between the two processes can be highlighted through this example. In the functioning of an engine, to accurately approximate the expansion taking place in an internal combustion engine as reversible, we would have to assume that the respective temperature and simultaneous pressure applied to alter uniformly. Further, this change shall be constant throughout the volume after the spark. So, if this happens, then the process is reversible. Obviously, this is not true, and there is a flame front and sometimes even engine knocking. So, in reality, there is some energy lost in the functioning of the engine fur to engine knocking and the flame front; thus, the process is irreversible.





