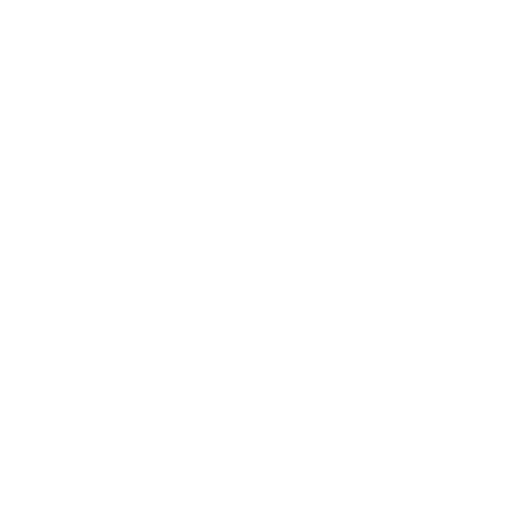

What is Boron Carbide?
Boron carbide (chemical formula B₄C) is a boron–carbon ceramic and covalent substance that is used in tank armour, bulletproof vests, engine sabotage powders, and a variety of other industrial applications. It is one of the hardest known materials, second only to cubic boron nitride and diamond, with a Vickers hardness >30 GPa.
Structure of Boron Carbide
[Image will be Uploaded Soon]
Boron Carbide Crystal Structure
Boron carbide has a complex crystal structure that is characteristic of borides centred on icosahedrons. B₁₂ icosahedra forms a rhombohedral lattice unit (space group: R3m, lattice constants: a = 0.56 nm and c = 1.212 nm) around a C-B-C chain in the unit cell's middle, and all carbon atoms bridge the neighbouring three icosahedra. The B₁₂ icosahedra and bridging carbons form a network plane that runs parallel to the c-plane and stacks along the c-axis, forming a layered structure. The B₁₂ icosahedron and the B6 octahedron are the two fundamental structural units of the lattice. The B₆ octahedra are too small to bind due to their small size. Instead, they bind to the B₁₂ icosahedra in the neighbouring layer, which weakens the c-plane bonding.
The chemical formula of "ideal" boron carbide is sometimes written as B₁₂C₃, and the carbon deficiency of boron carbide is defined in terms of a combination of the B₁₂C₃ and B₁₂C units, due to the B12structural unit. Some research suggests that one or more carbon atoms can be incorporated into the boron icosahedra, resulting in formulas like B₁₁CCBC = B₄C at the carbon-heavy end of the stoichiometry, but formulas like B₁₂(CBB) = B₁₄C at the boron-rich end. "Boron carbide" is therefore a family of compounds of varying compositions, rather than a single compound. B₁₂(CBC) = B₆.₅C is a common intermediate that approximates a frequently found element ratio. The crystal symmetry of the B₄C composition and the nonmetallic electrical character of the B₁₃C₂ compositions are both determined by configurational disorder between boron and carbon atoms at different positions in the crystal, according to quantum mechanical calculations.
Properties of Boron Carbide
Physical Properties of Boron Carbide
The density of boron carbide is nearly 2.52 g/cm³.
The Melting Point of boron carbide is 2445 °C.
The hardness of boron carbide ranges between 2900 - 3580Kg / mm2(Knoop 100 g).
Fracture Toughness of boron carbide is 2.9 - 3.7 MPam\[^{-1/2}\].
The Young's Modulus of boron carbide is 450 - 470 GPa.
Electrical Conductivity at 25 °C of boron carbide is 140 S.
Thermal Conductivity at 25 °C is 30 - 42 W/m.K
Thermal neutron capture of boron carbide is 600 barn.
Chemical Properties of Boron Carbide
Boron carbide is a tough substance with high toughness (roughly 9.5 to 9.75 on the Mohs hardness scale), a large cross-section for neutron absorption (i.e. strong neutron shielding properties), and resistance to ionising radiation and most chemicals. Vickers hardness (38 GPa), Elastic Modulus (460 GPa), and fracture toughness (3.5 MPam\[^{-1/2}\]) are all similar to diamond's (1150 GPa and 5.3 MPam\[^{-1/2}\]).
Boron carbide, after diamond and cubic boron nitride, is the third hardest material identified as of 2015, earning it the moniker "black diamond."
Boron carbide is a semiconductor with hopping-type transport dominating its electronic properties. The energy bandgap is determined by both the composition and the degree of order. The bandgap is measured to be 2.09 eV, and the photoluminescence spectrum is complicated by several mid-bandgap states. The content is usually of the p-type.
Preparation of Boron Carbide
Boron carbide was first synthesised in 1899 by Henri Moissan in an electric arc furnace by reducing boron trioxide with carbon or magnesium in the presence of carbon. In the case of carbon, the reaction takes place at temperatures above B₄C melting point and results in the release of a significant amount of carbon monoxide. If magnesium is used, the reaction can be performed in a graphite crucible, with the magnesium byproducts being extracted by acid treatment.
2B₂C₃ + 7C → B₄C + 6CO
Boron with a purity of 95% can be made from the oxide by combining it with powdered magnesium:
B₂O₃ + 3Mg → 2B + 3MgO
By passing a mixture of BCl₃ or BBr₃ and hydrogen over a heated tantalum filament, high-purity boron (>99.9%) can be obtained:
2BBr₃(g) + 3H₂(g) → 2B(s) + 6HBr(g)
Reaction of Boron Carbide
The oxidation of boron carbide powder has been observed at temperatures as low as 250°C in the presence of water vapour and 450°C in the absence of water vapour. Water vapour removes the B₂O₃ oxidation substance at a rate faster than the oxidation rate below 550⁰ - 600⁰C. The presence of B₂O₃ on the B₄C surface inhibited H₂O oxidation but not air oxidation. The rate was found to have a linear relationship with the partial pressure of water. The activation energy for the water - B₄C reaction was 11 kcal/mole, while it was 45 kcal/mole for the air - B₄C reaction. Dry air oxidises at a slower rate than water vapour before it reaches 700 (for a 235 mm water partial pressure).
Mechanism of Reaction Bonded Boron Carbide
Air Oxidation:
B₄C + 4O₂ → 2B₂O₃(l) + CO₂
Water Oxidation:
B₄C + 8H₂O → 2B₂O(l) + CO₂ + 8H₂
B₄C + 6H₂O → 2B₂O(l) + C + 6H₂
B₂O₃ Transport:
B₂O₃ + H₂O → 2HBO₂(g)
History
Boron carbide was discovered as a by-product of metal boride reactions in the 19th century, but its chemical composition was unknown. The chemical composition was not determined until the 1930s, when it was determined to be B₄C. The material's exact 4:1 stoichiometry remained a point of contention, as it is still slightly carbon-deficient in this formula in nature, and X-ray crystallography reveals that its structure is highly complex, with a mixture of C-B-C chains and B₁₂ icosahedra.
These characteristics argued against a straightforward exact B₄C empirical formula. The chemical formula of "ideal" boron carbide is sometimes written as B₁₂C₃, and the carbon deficiency of boron carbide is defined in terms of a combination of the B₁₂C₃ and B₁₂ CBC units, due to the B₁₂ structural unit.
Uses of Boron Carbide
Padlocks
Personal and vehicle anti-ballistic armor plating
Boron carbide is also used as ballistic armour in combination with other materials (including body or personal armour). The material's combination of high hardness, high elastic modulus, and low density provides it with extraordinary unique stopping power against high-velocity projectiles.
Grit blasting nozzles
Because of its high wear and abrasion resistance, boron carbide is used as slurry pumping nozzles, grit blasting nozzles, and water jet cutters.
High-pressure water jet cutter nozzles
Scratch and wear-resistant coatings
Cutting tools and dying.
Boron carbide abrasive is a powder used in polishing and lapping applications, as well as a loose abrasive in cutting applications such as water jet cutting, due to its high hardness. It's also useful for polishing diamond tools.
Neutron absorber in nuclear reactors: The material's ability to absorb neutrons without producing long-lived radionuclides makes it appealing as a neutron absorbent in nuclear power plants. Boron carbide is used in nuclear applications such as insulation, control rods, and shut down pellets.
Metal matrix composites
High energy fuel for solid fuel ramjets
In brake linings of vehicles
Some Product of Boron Carbide
Boron carbide dressing stick
[Image will be Uploaded Soon]
Boron Carbide Ceramics
[Image will be Uploaded Soon]
Important Points Related to Boron Carbide
The formula of tetraboron carbide is B₄C.
The density of tetraboron carbide is 2.52 g/cm³.
The molar mass of boron carbide is 55.255 g/mol.
The shape of the crystal structure of the boron carbide is rhombohedral.
The Melting point of boron carbide is 2,763 °C (5,005 °F; 3,036 K)
B₄C Boron carbide is insoluble in polar compounds like water.
Did you know that
Boron carbide ceramic is a covalent substance that is used in tank armour, bulletproof vests, engine sabotage powders, and a variety of other industrial applications.
The form of boron steel used in today's automobiles is extremely powerful. The yield point of boron steel is about 1,350–1,400 N/mm² (196,000–203,000 psi). That's about four times the strength of standard high-strength steel.
FAQs on Boron Carbide
Question: Why is Boron Added to Steel?
Answer: The biggest advantage of adding boron to steel is hardenability, with even small quantities improving hardness dramatically. Because of these properties, boron-containing carbon steels are used in situations where the base steel meets the majority of the requirements, such as wear resistance, but the hardenability is insufficient.
Question: How Much Does Boron Carbide Cost?
Answer: Since the process of producing boron carbide requires a significant amount of energy and time, boron carbide products can be up to ten times more costly than others. Boron carbide ceramic is a less durable material that currently dominates the market.





