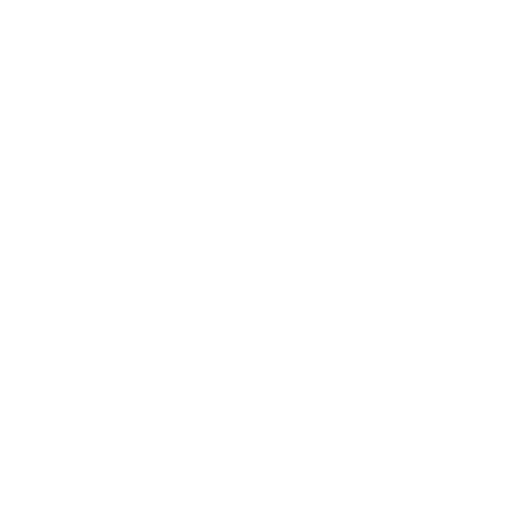

Alkenes - Member of Hydrocarbon Family
In organic chemistry, we study the various types of compounds formed from the carbon atom. Based on its bonding organic compounds are of three types Alkanes (single bond), Alkenes (double bonds), and Alkynes (triple bonds). When there is a single bond between the carbon-carbon atom then it forms alkanes (C-C). Whenever there is a double bond between the carbon-carbon atom then alkenes (C=C) are formed. In case if it shares a triple bond between carbon-carbon atom then it is alkynes (C≡C). A large number of pi bonds are present in alkanes that are closely held due to which they show a variety of chemical properties.
Chemical Property of Alkenes
Alkenes belong to the family of hydrocarbons containing a double bond between carbon-carbon atoms.
Alkenes are less stable than alkanes and more stable than alkynes.
Single bond > Double bond >Triple bond
Alkenes exist in all three solid-liquid and gaseous states.
Alkenes are less soluble in water due to weak Van-Der-Waal forces
The boiling point of alkenes depends on the molecular structure, the longer the molecular chain, the higher will be its boiling point.
Functional groups are responsible for the polarity of alkenes.
Reactions of Alkenes
Ozonolysis
Another significant chemical property of alkene compounds which, in the addition of ozone or three molecules, led to the formation of ozone at which upon reduction with zinc dust and water produces aldehydes and ketones respectively. This reaction is also considered a method of preparation for aldehydes and ketones.
Oxidation reaction and Ozonolysis are prominently exhibited in the chemical properties of alkenes.
Reactions
Alkenes are less stable than alkanes, but they are more reactive. The majority of alkene reactions involve additions to this pi bond, resulting in the formation of new single bonds. Because alkenes may engage in a wide range of processes, including polymerization and alkylation, they are used as feedstock in the petrochemical industry.
With the exception of ethylene, alkenes have two reactivity sites: the carbon-carbon pi-bond and the presence of allylic CH centres. The former predominates, while the allylic site is also significant.
Reactions to addition
Many additional reactions occur in alkenes, which occur by opening up the double-bond. The majority of these addition reactions follow the electrophilic addition mechanism. Hydrohalogenation, halogenation, halohydrin production, oxymercuration, hydroboration, dichlorocarbene addition, the Simmons–Smith reaction, catalytic hydrogenation, epoxidation, radical polymerization, and hydroxylation are some examples.
Hydrogenation and its associated hydro elementation
The equivalent alkanes are produced through the hydrogenation of alkenes. The reaction is sometimes performed under pressure and at high temperatures. Almost often, metallic catalysts are necessary. Platinum, nickel, and palladium are the most common metals used in industrial catalysts. The manufacture of margarine is a large-scale application.
Many more H-can X's can be added in addition to the insertion of H-H across the double bond. These procedures are frequently of significant commercial importance. The addition of H-SiR3, or hydrosilylation, is one example. This process produces organosilicon compounds. Another reaction is hydrocyanation, which occurs when H-CN is added across a double bond.
Hydration
Alcohols are produced through hydration, which is the addition of water across the double bond of alkenes. Phosphoric acid or sulfuric acid catalyses the process. This process is carried out on a large scale in order to manufacture synthetic ethanol.
CH₂ = CH₂ + H₂O → CH₃ - CH₂OH
Alkenes can also be transformed to alcohol by the oxymercuration–demercuration reaction, the hydroboration–oxidation reaction, or Mukaiyama hydration.
Halogenation
The addition of elemental bromine or chlorine to alkenes results in vicinal dibromo- and dichloro alkanes (1,2-dihalides or ethylene dihalides) in electrophilic halogenation. The decolourization of a bromine solution in water is used to detect the presence of alkenes:
CH₂ = CH₂ + Br₂ → BrCH₂ - CH₂Br
Related processes are also used to calculate the bromine and iodine numbers of a chemical or mixture as quantitative measurements of unsaturation.
Hydrohalogenation
The addition of hydrogen halides, such as HCl or HI, to alkenes, produces the equivalent haloalkanes:
CH₃ - CH = CH₂ + HI → CH₃ - CHI - CH₂ - H
If the two carbon atoms at the double bond are attached to a differing number of hydrogen atoms, the halogen preferentially occurs at the carbon with fewer hydrogen substituents. This pattern is referred to as Markovnikov's rule. The employment of radical initiators or other chemicals might result in the opposite product outcome. In the presence of different contaminants or even ambient oxygen, hydrobromic acid, in particular, is prone to generating radicals, resulting in a reversal of the Markovnikov result:
CH₃ - CH = CH₂ + HBr → CH₃ - CH - CH₂ - Br
Polymerization
Polymerization techniques use terminal alkenes as precursors to polymers. Some polymerizations are economically significant because they produce polymers polyethene and polypropylene. Although they contain no olefins, polymers derived from alkene are commonly referred to as polyolefins. Polymerization can occur through a variety of methods. Natural rubber is produced by conjugated dienes such as buta-1,3-diene and isoprene (2-methylbuta-1,3-diene).
Bonding and structure
Bonding
A carbon-carbon double bond is made up of two bonds: a sigma bond and a pi bond. This double bond is stronger than a single covalent bond (611 kJ/mol for C=C vs. 347 kJ/mol for C–C), but it is not twice as strong. The average bond length of a double bond is 1.33 (133 pm) compared to 1.53 for a normal C-C single bond.
Each carbon atom in the double bond forms sigma bonds with three additional atoms by using its three sp2 hybrid orbitals (the other carbon atom and two hydrogen atoms). The pi bond is formed by the unhybridized 2p atomic orbitals that lie perpendicular to the plane formed by the axes of the three sp2 hybrid orbitals. This bond is located outside of the main C–C axis, with half of it on one side of the molecule and the other on the other. The pi bond is substantially weaker than the sigma bond, with a strength of 65 kcal/mol.
Because breaking the alignment of the p orbitals on the two carbon atoms incurs an energy penalty, rotation about the carbon-carbon double bond is limited. As a result, cis and trans isomers interconvert so slowly that they may be handled freely at room temperature without isomerization.
For compounds with three or four distinct substituents, more complicated alkenes may be denoted using the E–Z notation (side groups). For example, in (Z)-but-2-ene (a.k.a. cis-2-butene), the two methyl groups occur on the same side of the double bond, but in (E)-but-2-ene (a.k.a. trans-2-butene), the methyl groups appear on opposing sides. Butene's two isomers have differing characteristics.
Shape
The molecular geometry of alkenes contains bond angles of around 120° around each carbon atom in a double bond, as predicted by the VSEPR model of electron pair repulsion. The angle may change due to steric strain caused by nonbonded interactions between functional groups linked to the double bond's carbon atoms. Propylene, for example, has a C–C–C bond angle of 123.9°.
Bredt's rule asserts that a double bond cannot develop at the bridgehead of a bridged ring system unless the rings are sufficiently massive.
Using Fawcett's definition of S as the total number of non-bridgehead atoms in the rings, bicyclic systems need S ≥7 while tricyclic systems require S ≥ 11.
Physical characteristics
Alkenes and alkanes have many physical features, such as being colourless, nonpolar, and flammable. The physical state is determined by molecular mass: the simplest alkenes (ethylene, propylene, and butene), like the equivalent saturated hydrocarbons, are gases at room temperature. Higher alkenes are waxy solids, while linear alkenes with five to sixteen carbon atoms are liquids. The melting point of solids rises with increasing molecular mass.
Alkenes have stronger odours than their comparable alkanes. The odour of ethylene is pleasant and musty. Cupric ion binding to olefin in the human olfactory receptor MOR244-3 is involved in the smell of alkenes (as well as thiols). Strained alkenes, in particular, norbornene and trans-cyclooctene, have strong, disagreeable scents, which is consistent with the stronger complexes they form with metal ions such as copper.
Focus on the features of alkenes and find out how they are unique from the rest. Learn how they form a family of compounds by showing similar reactions and other chemical properties.
FAQs on Chemical Properties of Alkenes
1.What is the addition of Halogens?
Vicinal dihalides are the byproducts of alkene reactions with halogens such as bromine and chlorine. Iodine is an exception since, under normal conditions, it does not display an additional reaction. The addition of bromine to an unsaturated site causes bromine solution to lose its reddish-orange colour in carbon tetrachloride solution. As a result, this reaction is employed to detect the existence of unsaturation in a solution. The electrophilic addition reaction is shown by the reaction of alkenes with halogens.
2.What is an elimination reaction?
The room elimination of alkyl halides, alcohols, and related chemicals is a key approach for alkene synthesis in the laboratory. The most prevalent mechanism for -elimination is the E2 or E1 mechanism, however -eliminations are also known.
For most alkene synthesis, the E2 mechanism provides a more reliable -elimination approach than the E1 mechanism. The majority of E2 eliminations begin with an alkyl halide or alkyl sulfonate ester (such as a tosylate or triflate). The reaction is known as dehydrohalogenation when an alkyl halide is employed.
The more substituted alkenes (those with fewer hydrogens connected to the C=C) tend to predominate in unsymmetrical products (see Zaitsev's rule). Dehydrohalogenation of alkyl halides and dehydration of alcohols are two prevalent ways of elimination reactions. A typical example is depicted below; notice that the H should be anti to the leaving group if feasible, even if this results in the less stable Z-isomer.
3.Explain Hydrogenation and related hydro eliminations.
Hydrogenation and its associated hydro elementation-
The equivalent alkanes are produced through the hydrogenation of alkenes. The reaction is sometimes performed under pressure and at high temperatures. Almost often, metallic catalysts are necessary. Platinum, nickel, and palladium are the most common metals used in industrial catalysts. The manufacture of margarine is a large-scale application.
Many more H-can X's can be added in addition to the insertion of H-H across the double bond. These procedures are frequently of significant commercial importance. The addition of H-SiR3, or hydrosilylation, is one example. This process produces organosilicon compounds. Another reaction is hydrocyanation, which occurs when H-CN is added across a double bond.
4.Why are Alkenes Important Chemicals?
Alkenes are important compounds as they are used in diverse applications by the industries. They are used for the synthesis of alcohol, plastics, fuels, and detergents. There are many alkenes which are important among which ethene, propene, and 1,3-butadiene are the most important ones.
Ethene is used for the production of crude oil and natural gases. It is also used as feedstock for chemical products such as vinyl chloride and ethanol.
Propane is used for the production of oxidation products such as acrylic acids, glycerol, ester, etc.
1,3-butadiene is used for the synthesis of various types of synthetic rubbers.
5.Why are Alkenes More Reactive than Alkanes?
Alkenes are the compounds made up of double bonds between carbon atoms with loosely held pi bonds. Alkenes are relatively stable compounds but more reactive than alkanes as pi bonds are stronger than sigma bonds. Alkenes belong to the family of hydrocarbons containing a double bond. Most alkenes reactions involve the addition of a pi bond to obtain a new single bond compound. Whereas alkanes have single bonds and sp3-sp3 hybridization with sigma bonds. Sigma bond requires more energy for the action that’s why it is less reactive than alkenes compounds.





