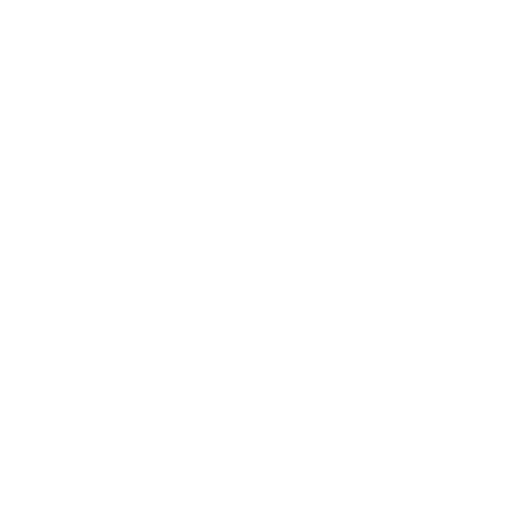

What Is Iron?
\[Fe_{3}O_{4}\], the chemical name Iron (II, III) oxide, occurs as a mineral. \[Fe_{3}O_{4}\] is a rare mineral that also occurs naturally as the mineral known as hematite. The mineral is a brownish to greyish or black stone, iron-like structure, also known as Magnetite or Magnetic oxide. It holds a characteristic metallic or a greasy lustre and is not transparent.
It comprises both \[Fe^{2+}\] and \[Fe^{3+}\] ions and is at times formulated as \[\textrm{FeO}\] ∙ \[Fe_{3}O_{4}\].
A Brief On \[Fe_{3}O_{4}\] Structure
Iron (II, III) oxide holds a cubic inverse spinel group structure. The \[Fe_{3}O_{4}\] structure comprises a cubic system, a close-packed array of oxide ions, in which all of the \[Fe^{2+}\] ions are present on one-half of the octahedral sites. At the same time, the \[Fe^{3+}\] are split evenly across the remaining tetrahedral sites and octahedral sites.
Both \[\gamma\] - \[Fe_{3}O_{3}\] and \[\textrm{FeO}\] hold a similar cubic structure with a close packed array of oxide ions. This packing leads to ready interchangeability between the three compounds through the oxidation and reduction process—these reactions leave a relatively small change to the structure of the compound.
(Image will be uploaded soon)
Iron (II, III) oxide exhibits ferrimagnetism due to the electron spins of the \[\textrm{Fe}\]-II and \[\textrm{Fe}\]-III ions. As a result, these ions undergo coupling in the octahedral sites, and the reels of the \[\textrm{Fe}\]-III ions present in the tetrahedral sites remain coupled but are anti-parallel to the former.
The total effect of the overall process is that the magnetic contributions of both sets of ions are not balanced, thus leading to a permanent magnetism.
Experimentally constrained models to display that the iron ions remain coordinated to the five oxygen ions on average when in the molten state. Furthermore, there is a distribution of coordination sites in the liquid form. The minority populations of both 4- and 6-fold coordinated Iron, and most \[\textrm{Fe}\]-II and \[\textrm{Fe}\]-III are 5-coordinated to oxygen.
What Is the \[Fe_{3}O_{4}\] chemical name?
\[Fe_{3}O_{4}\] is the chemical name of a compound called Magnetite, which is the mineral of Iron. The mineral holds the most magnetic property of all the naturally-occurring minerals occurring on Earth. The \[Fe_{3}O_{4}\] chemical name of the mineral is Iron(ll, lll) oxide as per IUPAC is known as ferrous-ferric oxide.
\[Fe_{3}O_{4}\] reaction
Iron (ll, lll) oxide is obtained through a series of chemical reactions-
Steel production process: The steel production process is a process that occurs through the reduction of the magnetite ore by carbon monoxide. This reaction takes place in a blast furnace and gives the following response:
\[Fe_{3}O_{4}\] + \[4CO\] \[\rightarrow\] \[\textrm{3Fe}\] + \[4CO_{2}\]
Production of the brown pigment: Brown pigment is a by-product that occurs when the mineral magnetite undergoes controlled oxidation. This reaction leads to the production of quality γ-\[Fe_{3}O_{4}\]. The reaction takes place as follows:
\[2Fe_{3}O_{4}\] + \[\frac{1}{2}O_{2}\] \[\rightarrow\] \[3\gamma\] \[Fe_{3}O_{4}\]
(Magnetite) (Maghemite)
Production of the red pigment: Red pigment is a by-product that occurs when the Magnetite undergoes vigorous calcining. This reaction leads to the production of a quality α-\[Fe_{3}O_{4}\]. The reaction takes place as follows:
\[2Fe_{3}O_{4}\] + \[\frac{1}{2}O_{2}\] → 3 \[\alpha\] - \[Fe_{3}O_{3}\]
(Magnetite) (Hematite)
Introduction To \[Fe_{3}O_{4}\] Nanoparticles
Iron oxide nanoparticles are iron oxide particles that differ in diameters between about one and 100 nanometers. Magnetite occurs in two predominant forms, and the oxidized form of the mineral is called maghemite \[(\gamma - Fe_{3}O_{3})\].
Iron oxide nanoparticles hold superparamagnetic properties that have drawn extensive interest leading to potential applications in multiple fields.
Iron oxide nanoparticles, ferrimagnetic maghemite, comprise \[Fe^{3+}\] vacancies, while the ferrimagnetic Magnetite contains \[Fe^{2+}\] and \[Fe^{3+}\] vacancies.
The composition of these two elements gives the compound biocompatibility, biodegradability, and the possibility to tailor magnetic behaviour, leading to an extensive application in medicine.
However, the nanoparticle size, aggregation, electronic, and magnetic properties significantly influence the biological effect of the compound.
The magnetic targeting iron nanoparticles commonly serve as a podium for attaching drugs like doxorubicin (DOX). However, their primary application is in tumour therapy, resulting in oxidative stress and hyperthermia, leading to tumour cell damage.
Synthesis Of Iron Oxide Nanoparticles
The preparation process of Iron oxide nanoparticles significantly affects the size, distribution, shape, and surface chemistry of the particles. The nanoparticles also determine to a great extent the type of structural defects or impurities, or distribution in the nanoparticles.
However, multiple factors affect magnetic behaviour. As a result, there are many recent attempts to develop techniques and processes that yield "monodisperse colloids" consisting of nanoparticles in a structured uniform in size and shape.
Coprecipitation
The employed method, by far, is coprecipitation, which is of two types. The first batch of ferrous hydroxide suspensions undergoes partial oxidation with different oxidizing agents. For example, the spherical magnetite particles have a narrow size distribution consisting of mean diameters ranging from 30 to 100 nm. This process can be obtained from a Fe(II) salt, a mild oxidant (nitrate ions), and a base.
The other common method for this process occurs through the ageing stoichiometric mixtures of ferrous and ferric hydroxides in aqueous media. This process leads to the yielding spherical magnetite particles that are homogeneous in size. In this method, the following chemical reaction occurs as follows-
\[2Fe^{3+}\] + \[Fe^{2+}\] + \[8OH\] → \[Fe_{3}O_{4}\] + \[4H_{2}O\]
The optimum conditions for this reaction occur between a pH 8 and 14, with the \[Fe^{3+}\] or \[Fe^{2+}\]
ratio being 2:1 and a non-oxidizing environment. This reaction is highly susceptible to oxidation. Thus the compound magnetite gets transformed to maghemite in the presence of oxygen.
\[2Fe_{3}O_{4}\] + \[O_{2}\] → \[2\gamma\] \[Fe_{3}O_{3}\]
The shape and size of the Iron oxide nanoparticles can be manually controlled by adjusting physical factors such as the nature of the salts (perchlorates, chlorides, sulfates, and nitrates), pH, temperature, ionic strength, or the \[\frac{Fe(II)}{Fe(III)}\] concentration ratio.
Microemulsions
A microemulsion is a stable isotropic dispersion of two immiscible liquids. Microemulsions consist of nanosized domains of either one or both liquids of a stabilized interfacial film medium of surface-active molecules.
Microemulsions are further categorized as oil-in-water or water-in-oil, depending on the continuous and dispersed phases. Water-in-oil is the most popular combo for the synthesis action of many kinds of nanoparticles.
The water and oil components are mixed with an amphiphilic surfactant that lowers the surface tension between water and oil, thus, making the solution transparent. The water nanodroplets in this process act as nanoreactors for synthesizing nanoparticles.
The shape of the water pool is spherical, and the nanoparticles' size also depends on the size of the water pool, which enables the size of spherical nanoparticles to be tuned as per the size change of the water pool.
High-temperature decomposition of organic precursors
In the presence of hot organic surfactants, iron precursors lead to deterioration resulting in samples that hold a reasonable size control, good crystallinity, and narrow size distribution (5-12 nm), due to which the nanoparticles are easily dispersed.
The size of the particles plays a crucial role in instances of biomedical applications like magnetic resonance imaging, magnetic cell separation or magnetorelaxometry, and the magnetic nanoparticles produced by the method of high-temperature decomposition of organic precursors are very useful.
The most viable iron precursors in organic solvents include \[Fe (CUP)_{3}\], \[Fe (CO)_{5}\], or \[Fe (acac)_{3}\] that consists of surfactant molecules. A combination of Xylenes and Sodium Dodecylbenzene Sulfonate used as a surfactant commonly creates nanoreactors that lead to well-dispersed Iron (II) and Iron (III) salts to react.
FAQs on Iron
1.What are the various applications of \[Fe_{3}O_{4}\] nanoparticles?
The applications of Iron (II, III) oxide nanoparticles are most commonly in sections such as high-sensitivity biomolecular magnetic resonance imaging, catalysis, gene delivery for various therapeutics and medical diagnosis, superparamagnetic relaxometry, magnetic fluid hyperthermia, terabit magnetic storage devices, and magnetic particle imaging.
Another common application of Iron (II, III) oxide is its use as a black pigment, also known as Mars Black, in acrylic paint.
The mineral is a common ingredient in thermite which is commonly used for cutting steel.
The application of \[Fe_{3}O_{4}\] nanoparticles requires regular coating by agents such as alkyl-substituted amines, long-chain fatty acids, and diols. Due to this, they are commonly used in formulations for supplementation.
Iron (II, III) oxide nanoparticles help to protect steel from the process of rusting.
Another common application of Iron (II, III) oxide is in water-gas shift reaction and the Haber process as a catalyst.
2. What is \[Fe_{3}O_{4}\] and state a few of its Biomedical applications?
\[Fe_{3}O_{4}\], is a mineral that occurs as a rare mineral naturally known as hematite. It comprises both \[Fe^{2+}\] and \[Fe^{3+}\] ions.
Both maghemite and Magnetite are highly favoured in biomedical applications as they are highly biocompatible and are non-toxic to human beings. In addition, iron Oxide is highly and easily degradable and is very useful for in vivo applications.
The solubility of the compound was found to influence the cytotoxic response strongly.
A nanoparticle specific cytotoxic mechanism for uncoated iron oxide resulted from an experiment from the exposure of a human mesothelial cell line and a murine fibroblast cell line.

















