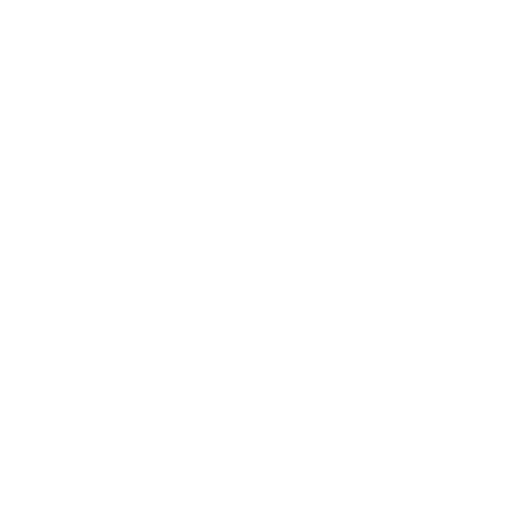

What is Meant by X-rays?
An X-ray, also known as X-radiation, is a type of high-energy electromagnetic radiation that penetrates the body. X rays consist of a packet of electromagnetic energy (photon) that originates from the electron cloud of an atom. The wavelength of most X-rays is in the range of 10 picometers to 10 nanometers, corresponding to frequencies of 30 petahertz to 30 exahertz and energies of 124 eV to 124 keV.
X-ray wavelengths are shorter than UV wavelengths and often longer than gamma-ray wavelengths. X-rays are known as Rontgen radiation in several languages, after the German physicist Wilhelm Conrad Rontgen, who discovered it on November 8, 1895. Now, let us go through the production and detection of x rays in brief.
How are X Rays Produced?
X-rays are formed when charged particles (electrons or ions) with sufficient energy collide with a substance.
[Image will be Uploaded Soon]
Production by Electrons
An X-ray tube or a vacuum tube that uses a high voltage to accelerate electrons emitted by a hot cathode to a high velocity can produce X ray electrons. The X-rays are produced when high-velocity electrons hit with a metal target, the anode. The target in medical X-ray tubes is normally tungsten or a more crack-resistant alloy of rhenium (5%) and tungsten (95%), however, molybdenum is occasionally used for more specific purposes, such as when softer X-rays are required, as in mammography. A copper target is the most common in crystallography, with cobalt being employed where fluorescence from the sample's iron concentration would otherwise be an issue.
The energy of the input electron, which is equal to the voltage on the tube times the electron charge, limits the maximum energy of the produced X-ray photon, hence an 80 kV tube cannot produce X-rays with an energy of more than 80 keV. When electrons collide with their target, two atomic processes produce X-rays:
If the electron has enough energy, it can knock an orbital electron out of the target atom's inner electron shell, causing characteristic X-ray emission (X-ray electroluminescence). The vacancies are then filled with electrons from higher energy levels, resulting in the emission of X-ray photons. This technique generates an X-ray emission spectrum with a few distinct frequencies, known as spectral lines. These are usually transitions from the top shells to the K shell (referred to as K lines), the L shell (referred to as L lines), and so on. It is K if the transition is from 2p to 1s, and it is K if the transition is from 3p to 1s. Because the frequencies of these lines are dependent on the target's substance, they are referred to as characteristic lines. In diffraction experiments, the K line is preferred since it has a higher intensity than the K one. As a result, a filter is used to remove the K line. Typically, the filter is constructed of a metal with one less proton than the anode material (e.g., Ni filter for Cu anode or Nb filter for Mo anode).
Bremsstrahlung is the radiation emitted by electrons as they scatter in a strong electric field near nuclei with a high Z (proton number). The spectrum of these X-rays is continuous. The energy of incident electrons limits the frequency of bremsstrahlung.
As a result, a tube's output is made up of a continuous bremsstrahlung spectrum that falls off to zero at the tube voltage, as well as many spikes at the characteristic lines. The greatest energy of X-ray photons ranges from around 20 keV to 150 keV because the voltages used in diagnostic X-ray tubes range from around 20 kV to 150 kV.
Both of these X-ray production procedures are inefficient, with only around 1% of the electrical energy spent by the tube transformed into X-rays, and so the majority of the electrical energy consumed by the tube is wasted heat. The X-ray tube must be built to dissipate surplus heat when producing a usable flux of X-rays.
Production by Fast-moving Ions
Fast protons or other positive ions can also emit X-rays. Proton-induced X-ray emission, also known as particle-induced X ray emission, is a common analytical technique. The production cross-section at high energies is proportional to Z12Z2-4, where Z1 is the atomic number of the ion and Z2 is the atomic number of the target atom. The same reference provides a summary of these cross-sections.
Production in Lighting and Laboratory Discharges
Lightning can also emit X-rays in conjunction with terrestrial gamma-ray outbursts. The basic mechanism is the acceleration of electrons in lightning-related electric fields, which results in photon generation via Bremsstrahlung. This generates photons with energy ranging from a few keV to tens of MeV. X-rays with a typical energy of 160 keV have been seen in laboratory discharges with a gap size of approximately 1-meter length and a peak voltage of 1 MV. The collision of two streamers and the generation of high-energy run-away electrons could be one reason. Microscopical simulations, on the other hand, have revealed that the period of electric field amplification between two streamers is too brief to produce a large number of runaway electrons. Air disturbances in the area of streamers have recently been proposed as a means of facilitating the creation of runaway electrons and so X-rays from discharges.
Detection of X-ray
Rontgen utilized photographic film as one of the first X-ray detectors, and this basic approach is still widely employed in medical applications. X-ray photons ionise radiation-sensitive silver halide crystals in an emulsion on the film surface, initiating the exposure process; the following photochemical change of the affected crystals darkens the exposed area (see radiation measurement: Photographic emulsions).
While photographic techniques have advanced much since Röntgen's time and are still incredibly valuable for qualitative applications, they are not well suited for more quantitative studies of X-ray intensities and spectrum content. A lot of improved detection techniques have been developed. Incoming X-ray photons ionise atoms in a gas-filled volume in a Geiger-Müller tube, or Geiger counter. Collisions between freed electrons and neutral atoms cause more ionization, resulting in an avalanche of charged particles and a strong electrical pulse that may be easily observed. Detection techniques based on the ionisation of gas atoms are more advanced and can distinguish between X-rays of different intensities (see radiation measurement: Proportional counters). Other typical detection systems rely on X-rays' capacity to cause visible fluorescence in crystals and charge separation in semiconductors (see scintillation counter) (see radiation measurement: Semiconductor detectors).
Do you know?
What are the Properties of X-ray?
Photons from X-rays have enough energy to ionise atoms and break molecular bonds. As a result, it is classified as ionising radiation, which is hazardous to living tissue. Radiation sickness is caused by a large radiation dose given over a short period of time, whereas lesser doses can increase the risk of radiation-induced cancer. In medical imaging, the benefits of the examination often outweigh the increased cancer risk. The ionising property of X-rays can be used in cancer treatment to use radiation therapy to eliminate cancerous cells. It's also used for X-ray spectroscopy material characterization.
The oxygen absorption edge at 540 eV, the energy - 3 dependency of photoabsorption, and a leveling off at higher photon energies due to Compton scattering may all be seen in the attenuation length of X-rays in water. Hard X-rays (right half) have a four-order-of-magnitude longer attenuation length than soft X-rays (left half). Hard X-rays can pass through relatively thick objects with little absorption or scattering. As a result, X-rays are frequently utilized to visualize the interior of visually opaque things. Over the X ray source spectrum, the penetration depth varies by several orders of magnitude. This enables the photon energy to be changed for the application in order to ensure sufficient transmission through the object while maintaining adequate image contrast.
Because X-rays have considerably shorter wavelengths than visible light, they can probe structures that are much smaller than can be viewed with a regular microscope. This feature is utilized in X-ray microscopy and X-ray crystallography to determine the locations of atoms in crystals.
FAQs on Production and Detection of X Rays
1. How are X Rays Produced?
Answer. When fast-moving electrons meet and interact with the target anode, X-rays are created as a result of their sudden slowing. More than 99 percent of the electron energy is transformed into heat during this deceleration process, and less than 1% of the energy is transformed into x-rays.
2. Are X Rays Deflected by Magnetic Fields?
Answer. Since X-rays do not carry a charge, they are not deflected by electrons or magnetic fields. Because x rays consist of electromagnetic radiations, no electrical or magnetic forces can deflect them.





