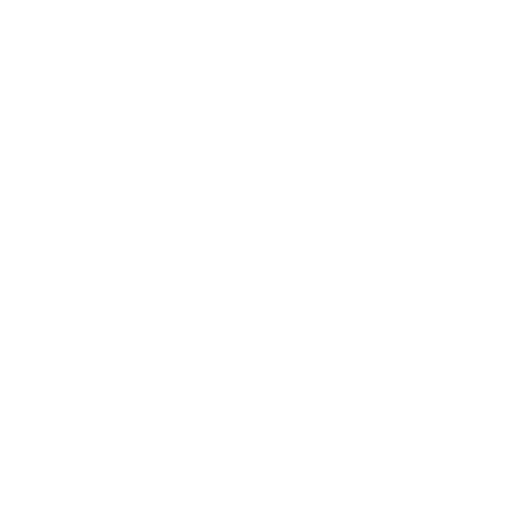

What is Semiconductor?
The semiconductor is a crystalline solid material, whose electrical conductivity lies between a conductor and an insulator. The semiconductors are mainly used in the manufacturing of electronic devices like capacitors, transistors, diodes, Integrated circuits, etc. Semiconductors are widely used in many devices for their reliability, compatibility, power efficiency and cost-efficiency. Semiconductors are also used in manufacturing batteries, optical sensors, light emitters, etc. The semiconductor also has a wide range of current and voltage handling capabilities. Semiconductor plays an important role in the communication system, signal processing, computing and system control applications in both domestic and industrial applications. This article explains the types of semiconductors, application of semiconductors, list of semiconductor materials and the electronic properties of semiconductors in detail.
(Image Will be Updated Soon)
Classification of Solid-State Materials
All the solid-state materials are mainly classified into three types, based on their electrical conductivity. They are listed below.
Insulator
Conductor
Semiconductor.
The insulator material never conducts electricity and it is in the order of 10-18 to 10-10 siemens per centimetre. Examples of insulators are glass, wood and quartz. The conductor material always conducts electricity and it is in the order of 104 to 106 siemens per centimetre. Examples of conductors are iron, aluminium, copper. The semiconductor material will act as an insulator still at a particular point and start to conduct after crossing the threshold limit. At the low temperature, some of the insulators, conductors and semiconductors may act as superconductors. Semiconductor examples are silicon and germanium. The electrical conductivity of semiconductors will vary depending on various factors like magnetic fields, temperature, illumination, and amounts of impurity atoms.
Origin of Semiconductor
In the early 19th century, people started to study semiconductor materials. The elemental semiconductors include germanium, silicon and tin, which are in column IV of the periodic table and selenium (Se) and tellurium (Te) in column VI of the periodic table. Pure silicon is one of the important materials that are used in the manufacturing of integrated circuits.
Before the invention of bipolar transistors, that is before 1947, the semiconductors were only used in two-terminal devices like photodiode and rectifiers. During the 1950s the most commonly used semiconductor materials are germanium. Later, scientists switched over to other semiconductors because of high current leakage and temperature elevation. After the 1960s, electronic industries started to adopt silicon in a wide range because of its lower leakage currents and shows high-quality insulation. Later, silicon became a great source for the semiconductor industries.
Electronic Properties of Semiconductor Materials
The atoms in the semiconductor materials are arranged in a three-dimensional period fashion. The intrinsic semiconductor is the purest form of semiconductor and it will not have any impurities. Each silicon atom will have four electrons in its outermost orbit, which are bounded completely with a covalent bond. Here, the force between the electrons and nuclei is strong, so they cannot conduct electricity without any external force or energy. The bandgaps between semiconductors are ranges from 0.25eV to 2.5 eV. For example, the bandgap of silicon is 1.12eV and the bandgap of gallium arsenide is 1.42 eV. The bandgap of the insulator, diamond is 5.5 eV.
At a low temperature, the electrons in a semiconductor will bound together with the nuclei and it could not give electrical conduction. During high temperatures, the thermal vibration breaks the covalent bond and produces free electrons to conduct electricity. If the electron breaks its covalent bond, it created electron vacancy with that bond. The electrons from neighbouring atoms will try to fill the vacancy and shift the vacancy from one crystal site to another. So, the holes in the atoms takes responsible for carrying positive charges and the electrons take responsibility for carrying negative charges in the opposite direction. When the electric field is applied to the semiconductor, both the holes and electrons in the crystal move in opposite direction for producing the electric current.
The electrical conductivity of semiconductors completely depends on the number of free holes and electrons per unit volume of the material. The holes and electrons in the semiconductors have different velocities and different mobilities in an electric field. The mobility of the holes and electrons in the semiconductor will decrease with an increase in temperature. In the intrinsic semiconductor, the number of holes and electrons are equal. So, the electrical conductivity of intrinsic semiconductors is poor at room temperature. In the intrinsic silicon material, the electrons will move at a velocity of 1,500 centimetres per second under one volt per centimetre. But the mobility of holes usually stands at 500cm²/V.s.
(Image Will be Updated Soon)
The electrical conductivity of the intrinsic semiconductor can be increased by adding some impurities. The resultant semiconductor is known as an extrinsic semiconductor. The process of adding impurities to the semiconductor is called doping. For creating the semiconductor with electrons as the major carrier, the element with excess electrons can be added to the intrinsic semiconductor. The resultant semiconductor is known as an n-type semiconductor. For example, arsenic can be added to silicon atoms. Because arsenic has five electrons and silicon atom has four electrons. For creating the semiconductor with holes as the major carriers, the element with fewer electrons can be added with the intrinsic semiconductor. The resultant semiconductor is known as a p-type semiconductor. For example, boron can be added to silicon atoms. Here, boron has three electrons and silicon has four electrons.
(Image Will be Updated Soon)
The p-n Junction
Generally, the p-type semiconductor has holes as the major carriers and acts as acceptors. The n-type semiconductor has electrons as the major carriers and acts as the donor in the p-n junction. While p-type semiconductors and n-type semiconductors are created in a single plate, there will be no free charge carriers at its junction, which is known as the depletion layer. This depletion layer acts as an insulator.
When the forward bias is applied to the p-n junction, the majority of charge carriers on each side rush into the junction and increase the current flow across the junction. If the reverse bias is applied to the p-n junction, the leakage current remains very small until a critical voltage is reached, at which point the current suddenly increases. Here, the sudden increase in the current shows the junction breakdown. This p-n junction is basic for the p-n-p and n-p-n junctions.
(Image Will be Updated Soon)
Uses of Semiconductor
Semiconductors are widely used in manufacturing electronics devices like transistors, diodes, sensors, integrated circuits.
Semiconductors are widely used in all electronic devices, like mobile phones, digital cameras, communication devices, trains, ATMs, etc.
This article explained what is semiconductors, the classification of solid-state materials, types of semiconductors, semiconductor examples, and electronic properties of semiconductors in detail.
FAQs on Semiconductor
Q1. What is semiconductor? What are its uses?
Answer: A semiconductor is an intermediate crystalline solid and its conductivity is between a conductor and an insulator. Usually, the semiconductor is widely used in the manufacture of various electronic devices like integrated circuits, diodes, transistors and resistors.
Q2. What are the types of semiconductors?
Answer: Semiconductors are mainly classified into two types based on their purity. They are Intrinsic semiconductors and Extrinsic semiconductors.
Q3. What is semiconductor material?
Answer: The commonly known semiconductor materials are silicon, germanium and gallium arsenide. The semiconductor in periodic table is called as "metalloid staircase". Silicon is the most commonly used semiconductor material. Gallium arsenide is also used in a wide range for the manufacture of laser diode, solar cells, integrated circuits, etc.





