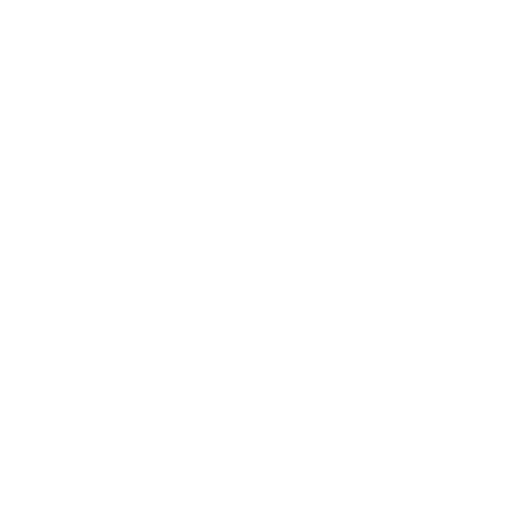

Chemical Bonding Molecular Orbitals of H₂
The procedure can be explained using the H₂ molecule as an example. The valence-shell orbitals of each hydrogen atom, which are the atoms' 1s orbitals, are used to build its molecular orbitals. These two orbitals can be superimposed in two ways: one by adding them together, and the other by subtracting them.
In the former, the amplitudes of the two atomic orbitals constructively interact with one another, resulting in an increased amplitude between the two nuclei. As a result, any electron occupying this molecular orbital is more likely to be found between the two nuclei, and its energy is lower than when it is restricted to either atomic orbital alone. A bonding orbital is thus described as a combination of atomic orbitals. It is also designated as an orbital and numbered 1 since it has cylindrical symmetry around the internuclear axis.
[Image will be Uploaded Soon]
Chemical Bonding Molecular Orbitals of H₂ and He₂
Since the MO created by subtracting the two 1s orbitals has cylindrical symmetry, it is also an orbital. Taking the difference between the two atomic orbitals, on the other hand, causes destructive interference in the internuclear field, where one orbital's amplitude is subtracted from the other. Since the destructive interference is complete on a plane halfway between the nuclei, there is a nodal plane—that is, a plane with zero amplitude—between them. Any electron occupying this orbital is not allowed to enter the internuclear field, and its energy is higher than if it occupied either atomic orbital. The orbital that forms in this way is known as an antibonding orbital, and it is sometimes abbreviated as * (or “sigma star”) or 2 since it is the second of the two orbitals.
The molecular orbital energy-level diagram for the H2 molecule displays the relative energies of molecular orbitals. The energies of the 1s orbitals of atoms A and B are shown on either side of the central ladder, and the energies of bonding and antibonding combinations are shown on the central two-rung ladder. The electrons are only added at this stage after the energy-level diagram has been set up. According to the Pauli exclusion theorem, each orbital may only have two electrons. Two electrons are present in H₂, and they join and fill the lower-energy bonding combination using the building-up theory. As a result, the molecule's electron configuration is 12 and the molecule's stability is determined by the occupation of the bonding combination. Its low energy is because of the accumulation of electron density in the internuclear region due to positive interference between the contributing atomic orbitals (at least according to the traditional interpretation).
Molecular Orbitals Energy
Many aspects of bonding can be explained by the Valence Bond Principle, but not all. We use another theory called the molecular orbital (MO) theory to supplement this one. The molecular orbital theory is a more advanced paradigm for comprehending chemical bonding.
New molecular orbitals are produced using a mathematical process called a linear combination of atomic orbitals in MO theory, which takes the concept of atomic orbitals overlapping to a new stage (LCAO).
Molecular orbitals are similar to atomic orbitals in many ways: – They are filled from lowest to highest energy (Aufbau principle).]
Atomic orbitals depict electron density in space associated with a specific atom, while molecular orbitals represent electron density in space associated with a specific molecule. The electron density is delocalized (spread out) over more than one atom as molecular orbitals are associated with the whole molecule.
Noble Gas Configuration
The noble gases are mentioned in the Periodic Table of Elements' rightmost column: helium, neon, argon, krypton, xenon, and radon. At room temperature, both of these elements are gaseous, colourless, odourless, and non-reactive with other elements. The outer, or valence, atomic orbitals are fully filled in the noble gases' electron configuration.
Electronic Configurations
Each element is identified by the number of positively charged protons in the nucleus and the number of electrons orbiting around it. Quantum mechanics identify the most likely orbital positions. Shells, subshells, and atomic orbitals are formed by these sites. Two electrons can be kept in the smallest atomic orbital, s. The next orbital, p, has a maximum capacity of six electrons. The lightest noble gas, helium, has just two electrons in its s orbital. Many of the remaining noble gases have absolute ‘s’ and ‘p’ orbitals in their outer shells. The "octet law" for noble gases states that each gas's valance (or outermost) shell has two s electrons and six p electrons. When a valance shell is fully filled, it cannot exchange electrons with other elements, resulting in gases that are too "noble" to mix with other atoms.
Pauli’s Exclusion Principle
In chemistry, the Pauli exclusion principle, along with Aufbau's Principle and Hund's Rule, is one of the most essential concepts. It is critical for students to understand, especially when studying electrons. It primarily aids in the comprehension of electron structures in atoms and molecules, as well as the classification of elements in the periodic table.
FAQs on Molecular Orbitals of H₂ and He₂
Q1. What is Meant by Bonding and Antibonding Molecular Orbitals? Give the Number of Electrons that Occupy the Bonding Orbital in H2+, H2, and He2.
Ans: Bonding Molecular orbital formed by constructive addition of wave functions has less energy and more stability. Anti-bonding molecular orbital is formed by destructive addition, has more energy, and less stability.
No. of electrons in bonding orbital of H is 1
No. of electrons in bonding orbital of H is 2
No. of electrons in bonding orbital of He is 2
Q2. What is the Pauli Exclusion Principle?
The Pauli exclusion principle states that no two electrons in a single atom can have the same set of quantum numbers (n, l, ml, and ms). To put it another way, each electron should have or be in its own distinct state (singlet state). The Pauli Exclusion Principle is based on two main principles:
Only two electrons may be in the same orbital at the same time.
The spins of the two electrons in the same orbital must be opposite or the orbital must be antiparallel.
The Pauli Exclusion Principle, on the other hand, does not only apply to electrons. It also refers to fermions and other half-integer spin particles. It is irrelevant for particles with an integer spin, such as bosons, since their wave functions are symmetric. Moreover, unlike fermions, bosons may share or have the same quantum states. Fermions are named after the Fermi–Dirac statistical distribution that they obey in terms of nomenclature. The Bose-Einstein distribution function, on the other hand, is where bosons get their name.

















