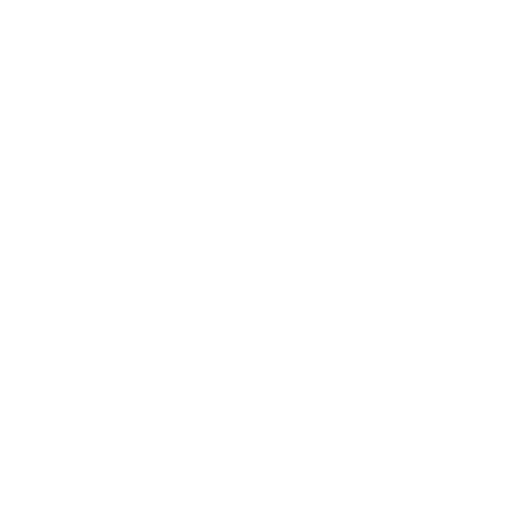

Shapes of Orbitals of an Atom
In chemistry, an orbital is a mathematical function which portrays the wave-like behaviour of an electron pair, electron or nucleons in Quantum Mechanics and Chemistry. Orbitals are also referred to as electron or atomic orbitals.
Atomic orbitals are the three- dimensional regions of space around the nucleus of an atom. Atomic orbitals allow the atoms to make covalent bonds. s, p, d and f orbitals are the most commonly filled orbitals. As defined by the Pauli Exclusion Principle, only two electrons can be found in any orbital space.
All the electrons which have the same value for n i.e the principal quantum number will be in the same shell. When the electrons share the same n, l and m they are said to be in the same orbital i.e. they have the same energy level, and they differ only in spin quantum number.
Nodes:
Node is a region where the probability of finding the electron will be zero. The nodal plane is the plane that passes through the nucleus on which the probability of finding an electron is zero. In an orbital, the number of nodal planes is equal to the azimuthal quantum number.
There are two types of nodes, they are angular and radial nodes. Angular nodes will be typically flat at fixed angles. Radial nodes are spheres at a fixed radius which occurs as the principal quantum number increases.
The total number of nodes of an orbital is the sum of angular and radial nodes and it is given in the terms of n and l quantum number and is given below:
N = n – l – 1
Types of Orbitals and Their Shapes
Atomic Orbitals can be classified into many types like s, p, d, f, g, h etc. But only the first four of the mentioned orbitals will be occupied on the ground state of an atom. Following are the explanation for the orbitals and their shapes:
The total values permitted form for a given value of I gives the number of orbitals of a type within a subshell. The four types of atomic orbitals match up to the values of l= 0, 1, 2 and 3. The orbitals with the value l = 0 are the s orbitals and they are spherically symmetrical in shape. It is with the greatest probability of finding the electron occurring at the nucleus.
The orbitals with the value l= 1 are the p orbitals which contain a nodal plane including the nucleus hence forming a dumbbell shape.
The orbitals with l= 2 are the d orbitals which have complex shapes with at least two nodal surfaces. The orbitals with l= 3 are called the f orbitals that are more complex.
Since the average distance from the nucleus will determine the energy of an electron, each atomic orbital with a given set of quantum numbers will have particular energy associated with it, which is called the orbital energy.
E = Z2/ n2 Rhc
The distribution of orbitals into their inner electronic core is called the penetration of orbitals. Example: The 2s orbital radial density is spread into the curve of 1s orbital. Same way, 3s orbital will be spread into 1s orbital and 2s orbital. Due to the spreading of electrons in 2s or 3s orbitals, it will not be screened fully by the inner 1s electrons from the nucleus. From s
orbitals to f orbitals, the extent of penetration decreases.
s > p > d > f
The above diagram denotes the penetration decrease from s to p orbitals as the radial distribution close to the nucleus for s is more when compared to p orbitals.
An ion or atom with one or more electrons occupies the higher energy orbitals and it is said to be in an excited state, whereas an ion or atom in which one or more electrons occupy low energy orbitals is said to be in its ground state.
The Shape of Orbitals
A large number of orbitals occupy an atom. If an orbital is smaller in size means that there is more possibility of finding the electron near to the nucleus. Same way, for the shape there is more possibility for finding electrons along certain directions rather than with the others.
The Shape of 1s Orbital:
The value of quantum numbers l and m are 0 for the s orbitals. The functions of Φ and Θ are independent of angles Φ and Θ for these values.
Each of the above mentioned two functions is equal to a constant term and for such orbitals, the equation will be
Ψ2n, 0, 0 α R2n, 0
Angular function of s orbitals: ΘΦ = (1/4π)½
For s orbitals, l = 0, the angular wave function is independent and constant of the angles Φ and θ. A2 is the probability of finding an electron at a specified direction Φ and Θ from nucleus to infinity at any distance.
For s orbital, the probability of finding the electron is maximum at r= 0 and it decreases exponentially with a distance r. The plots of R and R^2 are spherically symmetrical around the nucleus, so these plots exist around the nucleus.
The three- dimensional plots of ψ^2 Vs r are clear from the above diagram of the dot- population picture or boundary surface. In the above dot- population picture, the relative probability value at a given location is shown by the density dots near to it.
The dot population picture shows the actual description of the time average distribution of the electron. In the above diagram, the dot population picture R 2/ 1s Vs r is shown clearly.
In the equal probability contours, the contours can be drawn by joining the points of identical probability. For any of the s orbital, around the nucleus, these contours are spherically symmetrical.
The Shape of 2s Orbital:
For 2s orbital, it will be
Ψ 2/ 2, 0, 0 α R 2/ 2, 0
The dot population picture for 2s orbital which is shown above.
For the given value of r, the function of R might have positive value, zero or even negative value. The probability plots will always be positive as the square of a negative quantity or positive quantity is always positive.
From the above diagram, it is clear that for 2s orbital, there will be 2 maxima in the R 2/ 2 Vs r plot. It will be one at r= 0 and the other one at nearly 2= 210 pm, between these two maxima the probability becomes zero at about r= 105 pm. This is called the nodal point.
The size of the 2s orbital is larger than that of the 1s orbital. This is because the 2s orbital size resides farther away from the nucleus when compared to that of the 1s orbital.
The Shape of p Orbital:
Here, the quantum number m fixes the angular momentum direction. The quantum number also fixes the direction of the orbital in space.
Example: There are three orbitals of p orbitals (I= 1)
These values correspond to the three values of m(+1, 0, -1). The P0, P+1 + P-1 and P+1 – P-1 plots indicates the dumb-bell shaped orbitals. These are also perpendicular to each other pointing towards the x, y, and z-axis. For this reason, they are called px, py, pz orbitals.
The Shape of 2p Orbitals:
I= 1 for the p orbitals and there will be three orbitals for this type. These orbitals will correspond to three different values which are =1, 0, -1 of the magnetic quantum number called m.
Ψ^2 2, 1, 0 = R 2/ 2 θ^2 1, 0 Φ 2/ 0
Ψ^2 /2, 1+ 1 = R 2/ 2, 1 θ^2/ 1, 1 Φ 2/ 1
Ψ^2 /2, 1, -1 = R 2/ 2, 1 θ^2/ 1, 1 Φ 2/ -1
For all the three 2p orbitals, the R2, 1 Vs r and R2/ 2 Vs r plots are the same. This is because the function of R depends only on the quantum numbers I and n.
Unlike the 2s orbital, the 2p orbital the probability will be minimum at the nucleus and it has a maximum value of r= 104 pm. thereby, with the distance it decreases exponentially. The 2p orbitals will have directional characteristics which are due to the angular functions Φ and ʘ.
The Shape of d Orbital:
There are five d orbitals which are selected as "dx y, d y z, dx z", dx^2-y^2, dz^2. The energy of the d orbitals are equivalent but the shape of dz^2 orbitals is different from the other ones.
The Shape of 3d Orbitals:
The value of I= 2 for d orbitals and for I= 2 the five values of m are permissible. The values for the d type orbitals are +2, +1, 0, -1 and -2.
It is necessary to have the knowledge of the 3 d orbitals as it will be helpful in discussing the chemistry of many elements.the 3d orbitals can be classified into 2 categories. They are as follows:
1. Orbitals that have maximum probability distribution along with the 3dz^2 and 3d x^2-y^2 axes.
2. Orbitals that have a maximum probability distribution in between the two axes 3dxy, 3dyz and 3dxz.
F Orbital:
An f orbital has the secondary quantum number l = 3. There are seven f orbitals with ml = -3, -2, -1, 0, +1, +2, +3 and these orbitals are not occupied in the ground state until element 58 (cerium). XeXe 6s24f5d is the electronic configuration for cerium.
The f orbitals are deeply buried beneath the valence shell even for the elements beyond cerium. f orbitals have three nodal planes and it has complex shapes with the atomic nucleus at the centre.
The 4fy3- 3x2y orbital matches up to l = 3, ml = -3, and n = 4.
The 4fxyz orbital matches up to l = 3, ml = -2, and n = 4.
The 4f5yz2- yr2 orbital matches up to l = 3, ml = -1, and n = 4.
The 4f5z3- 3zr2 orbital matches up to l = 3, ml = 0, and n = 4.
The 4f5xz2- xr2 orbital matches up to l = 3, ml = +1, and n = 4.
The 4fzx3- xy2 orbital matches up to l = 3, ml = +2, and n = 4.
The 4fx3- 3xy2 orbital matches up to l = 3, ml = +3, and n = 4.
Biography of Robert S. Mulliken
Robert Sanderson Mulliken was born in 1896 in the United States. Lucia Maria (Mrs. John P. Heard) and Valerie Noè and their children. Mulliken earned a B.Sc. from the Massachusetts Institute of Technology in Cambridge, Mass., in 1917, and a Ph.D. from the University of Chicago in Chicago, Ill., in 1921. Mulliken has been fascinated with valence theory and molecular structure for a long time.
Following his earlier work on isotopes and diatomic band spectra, he developed a conceptual integration of molecule electronic states, based on the concept of molecular orbitals. Electronegativities, dipole moments, and valence-state energies were among the topics covered. Following that, he published a set of studies on the conceptual understanding of absolute intensities in electronic spectra, which led to analyses on conjugated organic molecules and the quantum-mechanical definition of hyperconjugation.
Research on quantum-mechanical concerns underpinning molecular-orbital theory, as well as the use of interatomic overlap integrals as bond energy metrics, followed. His most current work has focused on the structure and spectra of molecular complexes on the one hand, and the structure and spectra of hydrogen, helium, nitrogen, and other tiny elements on the other, expanding and enhancing prior work. The University of Stockholm in 1960 (Ph.D.); Marquette University in 1967 (Sc.D.); and Cambridge University in 1967 (Sc.D.).
FAQs on Shapes of Orbitals
1. Who coined the term orbital?
In the year 1932, Robert Mulliken coined the term "orbital" as an acronym for the one orbital wave function. However, Niels Bohr had claimed plausibly at least 19 years before Robert Mulliken that electrons could circle around a small nucleus with specific angular momentum, and Japanese physicist Hantaro Nagaoka presented an orbit-based explanation for electronic activity as early as in the year 1904. One of the driving reasons behind the creation of quantum mechanics was to explain the behaviour of these electron "orbits."
2. What is molecular orbital theory?
In chemistry, the molecular orbital theory is a quantum mechanics-based technique for characterising the electronic structure of molecules. Particles in a molecule are not ascribed to particular chemical interactions among atoms in molecular orbital theory but are instead viewed as moving under the influence of the atomic nuclei in the entire molecule. Particles in a molecule are not ascribed to particular chemical interactions among atoms in molecular orbital theory but are instead viewed as moving under the effect of the nucleons in the entire molecule. The spatial and kinetic features of electrons are described by quantum mechanics as molecular orbitals, which encircle two or more atoms in a molecule and include valence electrons between them. By representing the configurations of bonded electrons, the molecular orbitals as linear combinations of atomic orbitals, molecular orbital theory transformed the understanding of chemical bonding (LCAO). The Schrödinger equation is approximated by using the density functional theory (DFT) or Hartree–Fock (HF) models. The core ideas of quantum chemistry are molecular orbital theory and valence bond theory.
3. What is the mulliken’s approach?
Robert Mulliken (1896–1986) who won the Nobel Prize in Chemistry 1966 devised a technique for determining electronegativity after seeing that elements with high initial ionisation energy have strong negative electron affinities and gain electrons in chemical processes. The Mulliken scale essentially takes the balance of an element's ionisation potential and high electron to determine its electronegativity. The valence state of an element in a compound is denoted by the letters EA and IE (XM=IE+EA2).
4. What is the pauling and mulliken scale of electronegativity?
The Mulliken scale simply takes the mean of an element's ionisation potential and electron affinity to determine its electronegativity. The EA and IE relate to the element's valence state in a combination, not the atom's base state. The Pauling scale is a numerical scale of electronegativities based on bond-energy estimates for covalently linked atoms. The Mulliken figure is 2.8 times higher than Pauling's. Linus Pauling created the first electronegativity scale, with carbon having a number of 2.55 on a scale ranging from roughly 0.7 (an approximation for francium) to 2.20 (for hydrogen) to 3.98. (Fluorine).
5. What are the limitations of molecular orbital theory?
On the premise of bond arrangement, molecular theory explains whether a molecule will exist or not. The concept of bond order is insufficient to describe the formation of polyatomic compounds. It doesn't provide any information regarding molecular geometry or molecule shape, for example. According to the Molecular orbital theory, electrons are dispersed. This suggests they're dispersed throughout the molecule. The fundamental limitation of our study of MO theory is that we can only address diatomic molecules (molecules with only two atoms linked together), otherwise the theory becomes exceedingly complicated.





